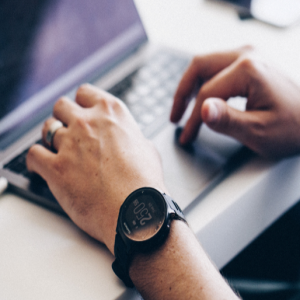
There is a never ending drive for improved performance in todays electronic devices, be they in, mobile, consumer, automotive or industrial applications. As a leading surface-finishing solutions provider, delivering chemistry, equipment, and service, Atotech is developing solutions to satisfy those demands.
Fan-Out Wafer-Level Packaging (FOWLP) was developed to enable higher performance and functionality, with increased reliability, and higher levels of integration in a smaller form factor, all while targeting significant cost reductions over exiting packing technologies.
Over recent years the “line and space” requirements have become increasingly more challenging, especially with regard to reliability and yield upon scaling. Through our dedicated research programs, Atotech has developed processes to meet these challenges, thus enabling extended scaling down to smaller feature sizes.
Fracture mechanism of thin RDL lines
In upcoming FOWLP designs, the mechanical properties of copper RDL lines become increasingly important, especially so with decreasing dimensions. During thermal load, there is a significant stress applied to RDL lines occurring at the transition from the die to the molding resin which is due to the mismatch in the coefficients of thermal expansion (CTE) of the respective materials.

Fig. 1: Schematic drawing of a FOWLP. The red lines highlight
the areas of high stress impact upon thermal load.
Stress upon thermal load may lead to RDL copper cracking. During each temperature cycle, the CTE mismatch leads to repeated plastic deformation and eventually, cracks in the copper. Failure of the RDL was observed to be a brittle, intergranular fracture, with segregation of impurities to the grain boundaries leading to that embrittlement. Such embrittlement along the grain boundaries was observed for samples with relatively high impurity levels and small grains, in comparison to the layer thickness. Once the grain size approached that of the layer, a transition from brittle to ductile behavior was detected. Furthermore, grain size evolution during annealing was found to be a function of impurity incorporation into the copper deposit.
In addition to embrittlement, segregation of impurities to grain boundaries during annealing resulted in an accumulation of voids in this region (Fig. 2, right). Medium impurity levels may be obtained by deposition of copper using Electrolyte 1, which contains a common leveler additive. In contrast, no voids and large grains were observed for copper deposits of high purity (Fig. 2, left). The latter being obtained by deposition with Electrolyte 2.

Fig. 2: Schematic representation of copper deposits with
low (left) and high (right) impurity levels upon annealing.
Therefore, it is assumed that highest reliability for RDL structures of small dimensions should be obtained for pure copper deposits with large grains. Both, grain size and purity being determined by co-deposition of organic additives.
In the following paper, our approach to affect the reliability of thin RDL copper structures through control of impurity levels as a function of proper molecule design will be explained in detail.
Additive impact on reliability of electrodeposited copper in thin RDL lines
The impurity level of the copper deposit is the key parameter to control reliability and mechanical properties of the fine pitch RDL lines. Co-deposition of organic additives may lead to impurities within the metal layer. Organic additives are often polymer-based and consist of functional groups, which modify the copper deposition mechanism. Co-deposition is known to occur via a Cu(I)-accelerator-leveler complex. Correct molecule design may help to avoid such complex formation and should lead to significantly higher purity of the deposit, but does require in-depth knowledge of the effects of the various functional groups of the organic plating additives. Surface-enhanced Raman spectroscopy (SERS) has been proven as a suitable technique to investigate the mode of operation of additives under plating conditions.
The Raman intensity varies as a function of the applied potential (Fig. 3). The band at approx. 270 cm-1 represents the Cu-Cl- stretching mode. The intensity of this mode increases with increasing potential. If a common leveler additive is adsorbed via surface-confined chloride, a similar potential-dependent trend is expected. The relevant C-H stretching mode of a common leveler additive, which consists of functional groups A, also varies in the same potential range as investigated before in case of chloride. The normalized Raman peak intensities as a function of the applied potential for chloride and a leveler with functional groups A are given in Fig. 3. This comparison clearly reveals the correlation between the signal intensities of adsorbed chloride and common leveler. Thus, functional groups A allow adsorption to the chloride-covered copper surface, but they also favor adsorption of the leveler-based complex and, thereby, facilitate co-deposition.

Fig. 3: Background-corrected, normalized intensity
of the Cu-Cl- (black) and C-H (red, leveler with
functional groups A) stretching modes during anodic
scan.
Weakening of the adsorption of leveler-based complexes should lead to increasing purity of the copper deposit. Such weakening may be obtained by levelers with properly designed functional groups B, which do not interact with surface-confined chloride. The peak position of the Cu-Cl- stretching mode may be used as an indicator for interaction of the leveler with the surface.
Fig. 4 compares the Cu-Cl- stretch peak position in absence and presence of levelers consisting of different functional groups. A leveler with functional groups A result in a significant shift of the peak, while levelers with functional groups B, which are designed to exhibit weak interaction to surface-confined chloride, show no or a very weak shift.

Fig. 4: Peak positions of the Cu-Cl- stretching mode
in absence of leveler (black) and in the presence of a
leveler with functional groups A (blue) as well as a
leveler with specially designed functional groups B (red).
In addition to adsorption on the copper surface via chloride, interaction between leveler and accelerator is crucial for the formation of Cu(I)-leveler-accelerator complexes. Such interaction may be detected by careful examination of the C-SSulfonate stretching mode of the accelerator in absence and presence of leveler additives employing SERS. Addition of a common leveler additive leads to a significant shift of the peak. On the other hand, combination of the accelerator with a specially designed leveler consisting of functional groups C shows no peak shift, which indicates weak interaction.
Functional groups, that prevent formation of the complex, may also be used to partially displace the leveler from the surface by the accelerator. The accelerator is known to adsorb on the copper surface to form a co-adsorption layer with chloride. In this case, levelers with functional groups A may interact with both, chloride and accelerator. A leveler, which contains a certain amount of functional groups C should be partially displaced by the accelerator. This effect may be investigated employing electrochemical potential transient experiments. These experiments monitor potential changes upon injection of organic additives during deposition of copper. Fig. 5 shows such an experiment starting with an additive-free electrolyte (virgin-makeup solution, VMS). At time 1, a leveler, which contains a certain amount of functional groups C, was added and led to development of an overpotential (shift to more negative potentials). The accelerator was subsequently injected at time 2 and a decrease of the overpotential could be observed. This recovery indicates partial displacement of the leveler from the surface.

Fig. 5: Potential transient at -10 mA cm-2 and 1000 rpm upon injection of a leveler, which contains functional groups C, at 250 s (time 1) and accelerator at 500 s (time 2) to VMS (50 g L-1 cupric ions, 50 g L-1 sulfuric acid, and 50 ppm chloride).
All the experiments discussed within this section allow identification of suitable functional groups for the design of leveler additives, which target to yield pure copper deposits.
Impurity and microstructure analysis of copper deposits
Impurities in copper deposits were determined by means of dynamic secondary ion mass spectrometry (DSIMS).
Copper deposits prepared with Electrolyte 2 are of much higher purity in terms of sulfur than the ones prepared from Electrolyte 1. Electrolyte 2 contains a specifically designed leveler, while Electrolyte 1 contains a common leveler. As sulfur impurities were found to cause embrittlement of copper and lead to formation of voids after thermal load, it is assumed that this kind of impurity should directly impact the RDL reliability. Deposits prepared with Electrolyte 2 are almost as pure as layers prepared from additive-free VMS.
Fig. 6 depicts the microstructure of deposits obtained with Electrolyte 2. In accordance with the requirements in terms of purity and microstructure provided in Section II, the cross section through a 3µm RDL line shows that the grains are of similar dimension as the line itself.
Conclusion
Upon decreasing the dimensions of RDL copper lines in FOWLP to, or even beyond 5 µm, reliability issues may occur due to mechanical stress imparted by the different material properties of the package, namely different CTE. This stress during thermal load may cause failure of the copper conductor lines of the RDL.
The failure mechanism was found to be intergranular fracture along the grain boundaries of the RDL lines, presumably due to embrittlement induced by sulfur and chloride impurities, which segregate to the grain boundaries during annealing, leading to an accompanying accumulation of voids. Therefore, improved reliability of sub 5 µm copper lines is expected for deposits of high purity and large grains, both of which may be influenced to great extent by plating additives.
Sophisticated design of copolymer-based levelers with suitable functional groups allows for fine tuning of the deposit properties, which will address the increasing reliability demands of upcoming RDL structures in FOWLP. Selection of these functional groups requires in-depth understanding of the effect of each of these groups on the copper deposition mechanism, with SERS and electrochemistry being proven to offer excellent guidance for this selection.
A copper plating process based on a leveler, synthesized according to the guidelines derived in this work, has exhibited excellent performance in terms of purity and constitutes an ideal candidate for sub 5 µm fine-pitch RDL structures.
Authors